Antibiotic Resistance and Phage Therapy as an Alternative Antimicrobial Treatment
- Pre-Collegiate Global Health Review
- Oct 14, 2021
- 9 min read
Danielle Hyunjung Cho, Yongsan International School of Seoul, Seoul, Republic of Korea
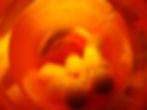
The Threat of Antibiotic Resistance
Since the discovery of antibiotics by Alexander Fleming in 1928, there have been concerns about the development of widespread resistance to these drugs. Fleming himself voiced these concerns when, in his 1945 Nobel acceptance speech, he warned about the potential danger of antibiotic-resistant pathogens (Fleming, 1945). Today, those hypothetical warnings are evolving into an alarming reality: antibiotic resistance is a growing threat that the World Health Organization (WHO) has named among "the world's most pressing public health problems" (WHO, 2019).

Figure 1. Tackling Drug-Resistant Infections Globally (O’Neill, 2016).
Figure 1 shows the number of deaths due to different causes recorded in 2016 (blue) and the estimated number of deaths due to antibiotic resistance (i.e. AMR) in 2050 (purple). The 2016 O’Neill Report predicts that the death toll due to antibiotic resistance will increase to a staggering 10 million people annually by 2050, surpassing cancer as the leading cause of death.
In addition to the loss of human lives, antibiotic resistance also comes with a significant economic cost. Treating six common types of multidrug-resistant microbial infections costs more than $4.6 billion annually in the U.S. alone (CDC, n.d.). Furthermore, by 2030, antibiotic resistance could force up to 24 million people into extreme poverty (UN, 2019).
Another consequence of antibiotic resistance is inequality in access to life-saving medical treatments. As is the case with all new medicines, new antibiotics are very expensive. As a result, developing countries and lower-income groups are at a significant disadvantage in accessing new antibiotics and other novel treatments for antibiotic-resistant bacterial infections (Nadimpalli et al., 2021). In developing countries, antibiotic shortages caused by inadequate funding and oversight of supply chains are a major issue (CDDEP, 2019; Pokharel et al., 2019). In Uganda, antibiotics are frequently not stored at the necessary temperature, so they are unusable even when they are available. Additionally, people may not be able to afford new antibiotics as they can cost “2 to 60 times the price of first-line medicines” (CDDEP, 2019). Currently, the situation is unfavorable towards lower-income groups, and this divide may only grow as the antibiotic resistance crisis continues.
A significant cause of the current antibiotic crisis is the abuse of antibiotics. Most obviously, overuse and misuse occur in medical settings, such as when people do not finish their prescription or antibiotics are prescribed without confirming whether the infection is caused by a bacterium or a virus (Fleming, 1945; Ventola, 2015). Less known, however, is the fact that abuse of antibiotics also occurs in agriculture where they are used to boost the growth of livestock and prevent bacterial infections in crowded, unsanitary factory farms (Salmond and Welch, 2008; Ventola, 2015).
Additionally, a new layer of the antibiotic overuse problem has come with the COVID-19 pandemic. There has been an increase in antibiotic use on COVID-19 patients (Ginsburg & Klugman, 2020; Knight et al., 2021). This trend is due to the difficulty in quickly differentiating between bacterial infections and viral infections when immediate treatment is required. Due to the urgency of the situation, doctors may use antibiotics before they have the chance to confirm that the infection is caused by a bacterium. Antibiotics are also used in prophylaxis to prevent further complications of a secondary bacterial infection. Due to these factors, approximately 70% of COVID-19 patients have received antibiotics (Knight et al., 2021). At the same time that this trend of increasing antibiotic use is concerning in terms of the long-run outlook on antibiotic resistance, the importance of using antibiotics to save human lives is undeniable. COVID-19 has complicated the issue of antibiotic overuse.
Another factor that has contributed to the worsening antibiotic resistance crisis is the sluggish development of new antibiotics. When antibiotics were first discovered, simply screening the soil was enough to find new antibiotics (von Bubnoff, 2006). Now, those methods only result in the same antibiotics that have already been discovered, so more difficult and expensive screening methods are needed (Hutchings et al., 2019; Plackett, 2020). While this complication in and of itself is enough to dissuade many pharmaceutical companies from developing new antibiotics, there is another problem: low profits. Unlike the medications one takes for their entire lifespan, antibiotics are only taken for the short time frame needed to eradicate the pathogen, and governmental agencies have placed roofs on their prices so that they remain relatively affordable.

Figure 2 . Graph of antibiotic and cancer drugs approved in the U.S. (Plackett, 2020).
Figure 2 highlights the worrying decline of new antibiotics production through a comparison of the number of new antibiotics and cancer drugs approved over the last four decades. While the approval of new cancer drugs has increased, the approval of new antibiotics has generally declined despite the growing threat from antibiotic-resistant pathogens.
Today, statements like “we are heading towards a post-antibiotic era” are becoming more and more common (Reardon, 2014). These concerns center around a future in which simple, commonplace surgeries (such as an appendectomy) could be death sentences (WHO, 2016). To avoid this future, pharmaceutical companies and governments must create public health campaigns and regulations to mitigate unnecessary usage of antibiotics, prioritize the prevention of bacterial infections through improved sanitation, and invest in the development of new drugs (O’Neill, 2016).
Phage Therapy, a Promising, Alternative Treatment
Scientific efforts to combat antibiotic resistance have centered around exploring alternative treatments to antibiotics. Phage therapy, which kills bacteria using phages (viruses of bacteria), is one such avenue that is currently being researched.
Despite the initial interest in phage therapy at the turn of the 20th century, it was largely sidelined after the discovery of antibiotics in 1928 (Lin et al., 2017). Over the past decade, interest in phage therapy surged once again, but research was stalled due to the lack of successful studies in humans. (“Interview with Robert Schooley”). Into this situation stepped Tom Patterson, a patient infected by the antibiotic-resistant pathogen Acinetobacter baumannii (Schooley et al., 2017; “Interview with Robert Schooley”). Although initially, a few antibiotics were still effective against the bacterial infection, the situation quickly worsened when, after a few months of treatment, the pathogen no longer responded to any antibiotics. Patterson was on the brink of death and out of treatment options. His wife, searching for alternative treatments, read about phage therapy and wanted to try it as a last resort. Three days after the first intravenous phage treatment, Patterson woke up from his coma. He has since recovered and is alive and well today.
Phage therapy has several advantages that make it a promising alternative treatment to antibiotics. One of these is its high specificity, which comes from the fact that phages can only infect a specific pathogen with a specific receptor. This characteristic is beneficial because it means that phage therapy does not harm the human microbiome, which is involved in a range of essential functions from synthesizing necessary metabolites to stimulating the immune system to absorbing nutrients (Krajmalnik-Brown et al., 2012; Loc-Carrillo & Abedon, 2011; Zheng et al., 2020). In contrast, broad-spectrum antibiotics can decimate these microbiomes, and consequently may have negative impacts on our health.
Another advantage of phage therapy is that there are many different kinds of phages that we can use for treatment. The astounding diversity of phages is illustrated by the fact that for every bacterial cell, there are approximately ten types of phages that use many different mechanisms to infect bacteria (Clokie et al., 2011). In addition to this diversity, phages are also extremely accessible: researchers can easily find them wherever bacteria are abundant. Thus, we can always find new types of phages for treatment even when bacteria develop resistance to the original phages used for treatment (Gordillo Altamirano & Barr, 2019).
As with any treatment, phage therapy also has limitations, including its risk of sepsis, its high specificity, and the limited amount of research that has been conducted on it so far. The risk of sepsis comes from the fact that, during phage preparation, bacterial components may contaminate the phages. If bacterial debris enters the bloodstream, the body can go into sepsis (whole-body inflammation), which may lead to organ failure (Caflisch et al., 2019; Hotchkiss et al., 2016). The second limitation--high specificity--is an advantage of phage therapy (as described earlier) but also a disadvantage because each phage can only target one type of bacteria. Due to this characteristic, treating infections caused by multiple bacteria requires many different types of phages (Gordillo Altamirano & Barr, 2019). Lastly, because research on phage therapy is still in its early stages, the interaction between phages and the human immune system is not completely understood (Nikolich & Filippov, 2020). However, these limitations do not negate the great potential of phage therapy as a treatment that can be used when all existing antibiotics lose their effectiveness against antibiotic-resistant bacteria.
To this last point, using phage therapy can also incapacitate antibiotic resistance on a small scale. The use of certain phages has been shown to cause a pathogen to lose its virulence or re-sensitize it to antibiotics (Kortright et al., 2019; Tagliaferri, 2019). This occurs because phages use specific surface structures to infect bacteria, which puts strong selective pressure on bacterial populations to evolve mutated versions or entirely absent versions of those surface structures. When these structures are essential for virulence or antibiotic resistance, these changes may result in reduced virulence and re-sensitization to antibiotics.
In the current landscape of antibiotic resistance, action is vital. To push against it and the disconcerting future it entails, we must make every effort to reduce the spread of antibiotic-resistant pathogens. In addition to these actions, researchers must continue to explore alternative treatments like phage therapy so that we can treat and potentially save people from life-threatening antibiotic-resistant bacterial infections.
References
Caflisch, K. M., Suh, G. A., & Patel, R. (2019). Biological challenges of phage therapy and proposed solutions: a literature review. Expert review of anti-infective therapy, 17(12), 1011–1041. https://doi.org/10.1080/14787210.2019.1694905
Centers for Disease Control and Prevention: CDC. (n.d.). 2019 AR Threats Report. Retrieved from https://www.cdc.gov/drugresistance/biggest-threats.html
Center for Disease Dynamics, Economics & Policy: CDDEP. (2019). Access barriers to antibiotics. https://cddep.org/wp-content/uploads/2019/04/AccessBarriersto Antibiotics_CDDEP_FINAL.pdf
Clokie, M. R., Millard, A. D., Letarov, A. V., & Heaphy, S. (2011). Phages in nature. Bacteriophage, 1(1), 31–45. https://doi.org/10.4161/bact.1.1.14942
Fleming, A. (1945). Penicillin. Retrieved from https://www.nobelprize.org/uploads/2018/06/ fleming-lecture.pdf
Ginsburg, A. S., & Klugman, K. P. (2020). COVID-19 pneumonia and the appropriate use of antibiotics. The Lancet. Global health, 8(12), e1453–e1454. https://doi.org/10.1016/S2214-109X(20)30444-7
Gordillo Altamirano, F. L., & Barr, J. J. (2019). Phage Therapy in the Postantibiotic Era. Clinical microbiology reviews, 32(2), e00066-18. https://doi.org/10.1128/CMR.00066-18
Hotchkiss, R. S., Moldawer, L. L., Opal, S. M., Reinhart, K., Turnbull, I. R., & Vincent, J. L. (2016). Sepsis and septic shock. Nature reviews. Disease primers, 2, 16045. https://doi.org/10.1038/nrdp.2016.45
Hutchings, M. I., Truman, A. W., & Wilkinson, B. (2019). Antibiotics: past, present and future. Current opinion in microbiology, 51, 72–80. https://doi.org/10.1016/j.mib.2019.10.008
Interview with Robert Schooley, Steffanie Strathdee and Tom Patterson. (n.d.). UC San Diego Health. https://health.ucsd.edu/news/topics/phage-therapy/Pages/Q-and-A.aspx
Knight, G. M., Glover, R. E., McQuaid, C. F., Olaru, I. D., Gallandat, K., Leclerc, Q. J., Fuller, N. M., Willcocks, S. J., Hasan, R., van Kleef, E., & Chandler, C. I. (2021). Antimicrobial resistance and COVID-19: Intersections and implications. eLife, 10, e64139. https://doi.org/10.7554/eLife.64139
Kortright, K. E., Chan, B. K., Koff, J. L., & Turner, P. E. (2019). Phage Therapy: A Renewed Approach to Combat Antibiotic-Resistant Bacteria. Cell host & microbe, 25(2), 219–232. https://doi.org/10.1016/j.chom.2019.01.014
Krajmalnik-Brown, R., Ilhan, Z. E., Kang, D. W., & DiBaise, J. K. (2012). Effects of gut microbes on nutrient absorption and energy regulation. Nutrition in clinical practice: official publication of the American Society for Parenteral and Enteral Nutrition, 27(2), 201–214. https://doi.org/10.1177/0884533611436116
Lin, D. M., Koskella, B., & Lin, H. C. (2017). Phage therapy: An alternative to antibiotics in the age of multi-drug resistance. World journal of gastrointestinal pharmacology and therapeutics, 8(3), 162–173. https://doi.org/10.4292/wjgpt. v8.i3.162
Loc-Carrillo, C., & Abedon, S. T. (2011). Pros and cons of phage therapy. Bacteriophage, 1(2), 111–114. https://doi.org/10.4161/bact.1.2.14590
Nadimpalli, M. L., Chan, C. W., & Doron, S. (2021). Antibiotic resistance: a call to action to prevent the next epidemic of inequality. Nature medicine, 27(2), 187–188. https://doi.org/10.1038/s41591-020-01201-9
Nikolich, M. P., & Filippov, A. A. (2020). Bacteriophage Therapy: Developments and Directions. Antibiotics (Basel, Switzerland), 9(3), 135. https://doi.org/10.3390/ antibiotics9030135
O’Neill, J. Tackling Drug-Resistant Infections Globally: Final Report and Recommendations. (2016, May). Review on Antimicrobial Resistance, Retrieved from https://amr-review.org/ sites/default/files/160518_Final%20paper_with%20cover.pdf
Plackett, B. (2020). Why big pharma has abandoned antibiotics. Nature, 586, S50-S52. doi: https://doi.org/10.1038/d41586-020-02884-3
Pokharel, S., Raut, S., & Adhikari, B. (2019). Tackling antimicrobial resistance in low-income and middle-income countries. BMJ global health, 4(6), e002104. https://doi.org/10.1136/bmjgh-2019-002104
Reardon, S. WHO warns against 'post-antibiotic' era. Nature (2014). https://doi.org/10.1038/nature.2014.15135
Salmond, G. P. C., & Welch, M. (2008). Antibiotic resistance: adaptive evolution. The Lancet, 372, S97–103. https://www.thelancet.com/pdfs/journals/lancet/ PIIS0140-6736(08)61888-7.pdf
Schooley, R. T., Biswas, B., Gill, J. J., Hernandez-Morales, A., Lancaster, J., Lessor, L., Barr, J. J., Reed, S. L., Rohwer, F., Benler, S., Segall, A. M., Taplitz, R., Smith, D. M., Kerr, K., Kumaraswamy, M., Nizet, V., Lin, L., McCauley, M. D., Strathdee, S. A., Benson, C. A., … Hamilton, T. (2017). Development and Use of Personalized Bacteriophage-Based Therapeutic Cocktails To Treat a Patient with a Disseminated Resistant Acinetobacter baumannii Infection. Antimicrobial agents and chemotherapy, 61(10), e00954-17. https://doi.org/10.1128/AAC.00954-17
Stone, E., Campbell, K., Grant, I., & McAuliffe, O. (2019). Understanding and Exploiting Phage-Host Interactions. Viruses, 11(6), 567. https://doi.org/10.3390/ v11060567
Strathdee, S. (2019, March 23). My husband was dying with a superbug. So I hunted down an experimental treatment to save him. Toronto Star. Retreived from https://www.thestar.com/news/world/2019/03/23/my-husband-was-dying-with-a-superbug-so-i-hunted-down-an-experimental-treatment-to-save-him.html
Tagliaferri, T. L., Jansen, M., & Horz, H. P. (2019). Fighting Pathogenic Bacteria on Two Fronts: Phages and Antibiotics as Combined Strategy. Frontiers in cellular and infection microbiology, 9, 22. https://doi.org/10.3389/fcimb.2019.00022
United Nations: UN. (2019, April 29). High-Level Interactive Dialogue on Antimicrobial Resistance. Retrieved from https://www.un.org/pga/75/antimicrobial-resistance/
Ventola C. L. (2015). The antibiotic resistance crisis: part 1: causes and threats. P & T : a peer-reviewed journal for formulary management, 40(4), 277–283. https://www.ncbi.nlm.nih.gov/pmc/articles/PMC4378521/
von Bubnoff A. (2006). Seeking new antibiotics in nature's backyard. Cell, 127(5), 867–869. https://doi.org/10.1016/j.cell.2006.11.021
World Health Organization: WHO. (2016, Nov. 3). WHO recommends 29 ways to stop surgical infections and avoid superbugs. Retrieved from https://www.who.int/ news/item/03-11-2016-who-recommends-29-ways-to-stop-surgical-infections-and-avoid-superbugs
World Health Organization: WHO. (2019, May 14). WHO Mission on Antimicrobial Stewardship and Quality Control Laboratory Network in Indonesia. Retrieved from https://www.who.int/indonesia/news/detail/14-05-2019-who-mission-on-antimicrobial-stewardship-and-quality-control-laboratory-network-in-indonesia
Zheng, D., Liwinski, T., & Elinav, E. (2020). Interaction between microbiota and immunity in health and disease. Cell research, 30(6), 492–506. https://doi.org/10.1038/s41422-020- 0332-7